Characterization and Sequencing of Duplex siRNA Using the BioAccord™ LC-MS System and Vion IMS QTof Mass Spectrometer in Combination With the waters_connect™ CONFIRM Sequence App
Abstract
Small interfering RNA (siRNA) has become a modality of great interest in pharmaceutical drug development due to its innovative use in gene therapy and cancer treatments over the last decade. With this growing interest, the demand for analytical solutions supporting its development, manufacture, and batch release have steadily increased. Chromatographic separation coupled with mass spectrometric detection has become state-of-the-art analytical methodology that has matured beyond application in product and process development into validatable assays that can be deployed into manufacturing and quality control laboratories.
Here, we present how column temperature plays a crucial role in controlling the interaction of nucleic acid duplexes during online chromatographic separations. By adjusting the column temperature, we can either preserve the duplex structure or denature the duplex into separate sense and antisense strands. Whilst this complementary approach is commonly used to assist in molecule identification and attribute monitoring, we show how it can also benefit duplex sequence confirmation. Studies with these duplex structures were conducted on both the BioAccord LC-MS System and the Vion IMS QTof LC-MS System.
Benefits
- Develop quality control methods with automated compliance-ready LC-MS workflows that are accessible to non-MS experts
- Assess duplex purity, duplex identity, and duplex sequence from a single analysis
- Facilitate fast and automated interpretation of complex tandem mass spectra (MS/MS) and data-independent MSE (no specific precursor ion selection) siRNA Duplex mass spectra using the CONFIRM Sequence App within the compliance ready waters_connect informatics platform
Introduction
Oligonucleotide drugs have emerged as valuable tools to treat hereditary and non-hereditary diseases across various classes.1–3 Silencing RNA (siRNA), which consists of duplex RNA molecules that can specifically bind to cellular mRNA and inhibit its function has emerged as a successful modality for disease treatment.4
Given the need for these therapeutics to precisely target the intended mRNA sequence and avoid secondary site effects, establishing an effective quality control procedure is essential to product quality, safety, and efficacy. Various methods are employed for characterization before advancing siRNA to clinical trials or as a drug product. Liquid chromatography techniques, including ion pairing reverse phase (IPRP), ion exchange (IEX), size exclusion (SEC), and hydrophilic interaction (HILIC), have been implemented.5,6,7 Coupling these techniques with mass spectrometry has become common, as mass spectra provide insights into co-eluting impurities and facilitates assignments of these product variants.8 High-resolution mass spectrometry with collision-induced dissociation (CID) is widely used to confirm the sequences of these molecules and localize sites of modification.9
Here, we introduce a routine LC-MS methodology employing ion-pairing reversed phase chromatography (IP-RP) coupled with data-independent (no precursor selection) MSE fragmentation for the detection and sequencing of siRNA duplexes. This method leverages automated data processing coupled with a highly accessible BioAccord LC-HRMS System, operating under waters_connect informatics, to support compliance-ready data acquisition, processing, and reporting.
The BioAccord LC-MS System (Figure 1) was introduced in 2019 as a compact, robust platform for routine biopharmaceutical analysis that is accessible to non-MS experts. The fully integrated BioAccord LC-MS System used here is comprised of an ACQUITY™ UPLC™ Premier I-Class PLUS System, a Tunable Ultraviolet (TUV) Optical Detector, and an ESI-TOF ACQUITY RDa™ Mass Detector. The sequencing results were then compared against targeted LC-MSMS analysis using the Vion IMS-QTof MS instrument.
Unlike the regular (unmodified) oligonucleotides, siRNA molecules can be challenging to characterize by LC-MS due to their complexity and stability. By controlling and adjusting column temperature we can induce duplex conformer dissociation into Sense and Antisense strands increasing MS response and decreasing spectral complexity. This allows for improved sequence coverage, strand identification, and purity to be evaluated from a single injection. Alternately, we can decrease column temperature to preserve duplex conformation on column, allowing identification, and purity to be measured at full duplex level. The combination of these two approaches within a single analysis were evaluated for their ability to provide insights into the quality attributes of the duplex siRNA molecule studied.
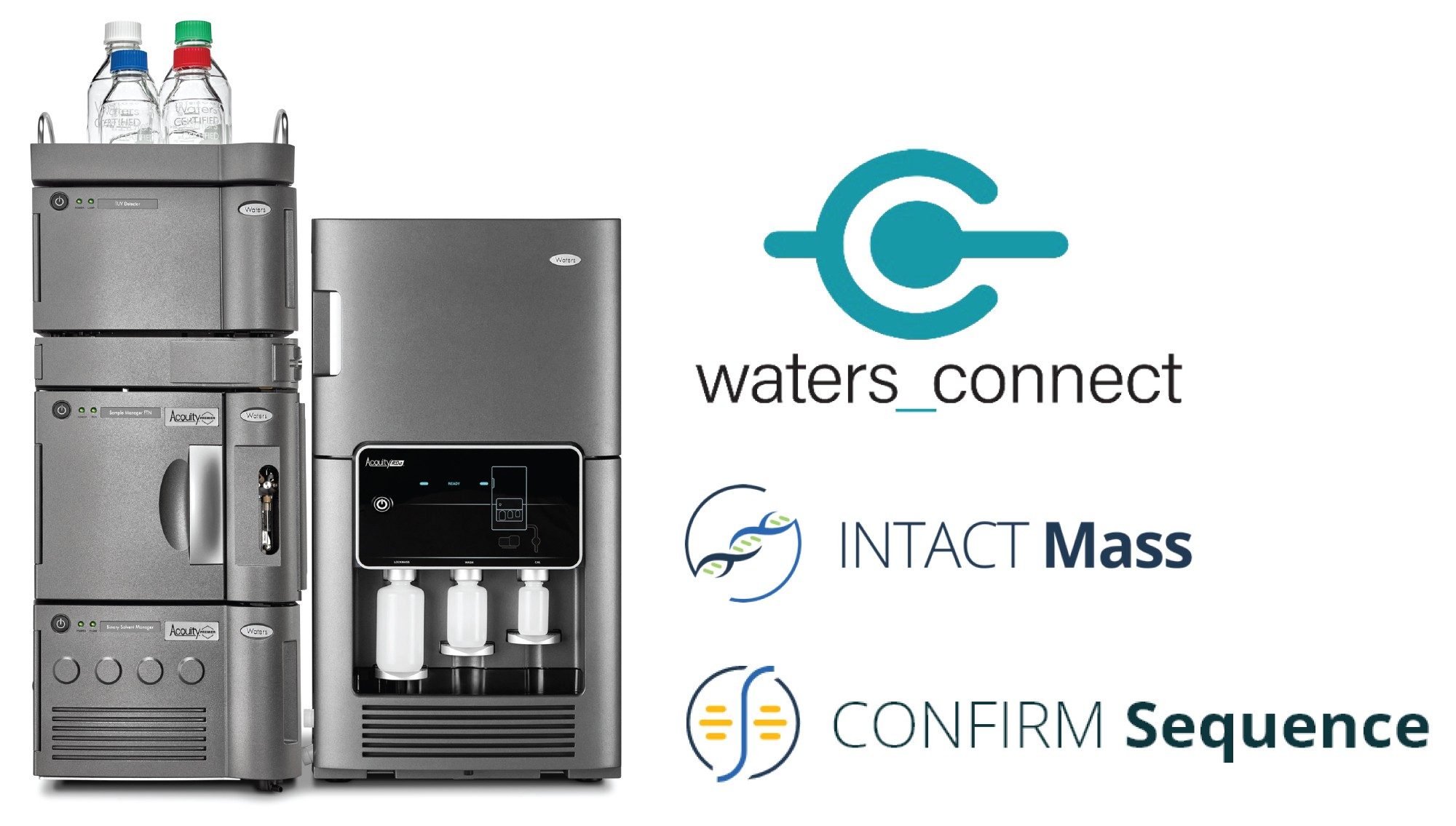
Experimental
Sample Description
Two RNA single strands, annealed in duplex, with the following sequences were used for the experiment:
Experimental Table
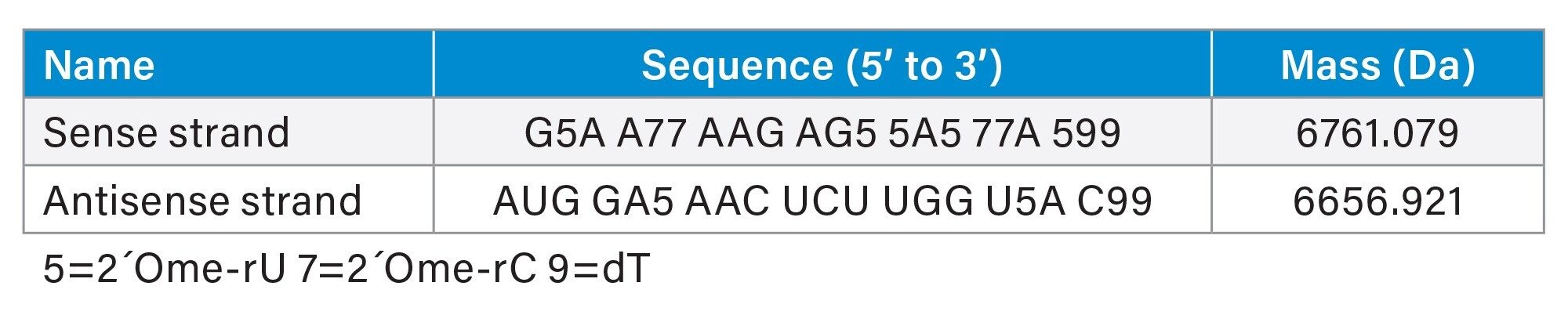
Hybridization: The single strands were pipetted in equimolar amounts and were heated to 75 °C and kept at this temperature for 5 minutes. Afterwards the solution was cooled down to 37 °C with a cooling rate of 1 °C/min.
The single strands and the duplex were then measured using 25 °C and 60 °C column temperature. (Tandem)-mass spectra were acquired in a targeted MS/MS acquisition (Vion IMS QTof) with fixed precursor m/z and fixed collision energy and using MSE data independent fragmentation acquisition (BioAccord System).
LC Conditions
LC system: |
Waters™ I-Class system (coupled to Vion IMS QTof or RDa-detector in a BioAccord System) |
Detection: |
UV 260 nm |
Vials: |
LCGC Certified Clear Glass 12 x 32 mm Screw Neck Vial, Total Recovery, with Cap and Preslit PTFE/Silicone Septum (p/n: 186000385C) |
Column(s): |
ACQUITY UPLC Oligonucleotide BEH, 130 Å, C18, 1.7 µm, 2.1 x 50 mm (p/n: 186009484) |
Column temperature: |
25.0 °C and 60.0 °C |
Sample temperature: |
6.0 °C |
Injection volume: |
1 µL |
Flow rate: |
0.300 ml/min |
Mobile phase A: |
7 mM TEA, 80 mM HFIP in aqueous solution |
Mobile phase B: |
3.5 mM TEA, 40 mM HFIP in 50% methanol |
Gradient Table

MS Conditions
MS system: |
Waters BioAccord LC-MS |
Mode: |
Full scan with fragmentation |
Mass range: |
Low (50–2000 m/z) |
Polarity: |
Negative |
Scan rate: |
10 Hz |
Cone voltage: |
40 V |
Fragmentation cone voltage: |
90–110 V |
MS system: |
Waters Vion IMS QTof |
Mode: |
MS/MS |
Mass range: |
50–2000 m/z |
Precursor: |
675.4 m/z for sense strand 664.9 m/z for antisense strand |
Scan time: |
0.5 s |
Collision energy |
20 V for both sense and antisense strand |
Results and Discussion
With the goal to streamline and efficiently evaluate duplex purity and identity, identify impurities, and confirm the correct sequences of both sense and antisense strands, we attempted to apply LC-MS/MS sequencing to denatured and nondenatured duplex siRNA oligonucleotides in a single analytical analysis. BioAccord LC-MS data were acquired in full scan MSE data-independent fragmentation mode and was acquired and processed on the waters_connect informatics platform. Automated spectral deconvolution was performed in the waters_connect INTACT Mass App using the combined ESI-MS spectra under the TIC peak to obtain an intact oligonucleotide mass measurement for strand identification, purity assessment, and modification localization. Oligonucleotide sequence analysis was then conducted using the waters_connect CONFIRM Sequence App. Finally, Vion IMS QTof data were collected in a targeted MS/MS mode to provide comparison against the BioAccord data-independent acquisition (DIA) sequence results.
Experiments conducted using LC column temperatures of 25 °C (non-duplex denaturing) and 60 °C (denaturing) using the BioAccord System or Vion IMS QTof mass detection demonstrate how these instruments can assess various parameters of double stranded oligonucleotides in a single analytical run.
At a column temperature of 25 °C, the duplex siRNA molecule is preserved on column (see Figure 2). Individual samples of the sense and antisense strands are chromatographically resolved from each other and the duplex molecule. While the moderate temperature maintains the duplex molecule’s intact structure throughout the LC analysis, the use of ion pairing reagents and low pH result in the denaturing of the complex upon electrospray ionization. This enables duplex spectra to be reviewed and characterized as individual strands (Figure 3A).
The data were processed through the automated waters_connect INTACT Mass App which enables scientists to analyze both shorter and longer oligonucleotides that supports processing capabilities such as TIC and UV peak detection, BayesSpray charge deconvolution, modification, and product-related impurity assignments and purity calculations for streamlined nucleic acid analysis without requiring significant user interventions. (Figures 3B and 3C).
The INTACT Mass application results (Figure 3C) facilitated the assignment of deconvoluted species co-eluting under the duplex chromatographic peak at 5.13 minutes. Due to this co-elution, the LC-UV purity is reported as 90% for all species. The INTACT Mass App also calculated the MS purity by considering all assigned co-eluting species as the product of LC and MS relative responses (LCxMS). Low ppm (<10 ppm) mass errors were observed for all deconvoluted identifications for both major and minor intensity species.
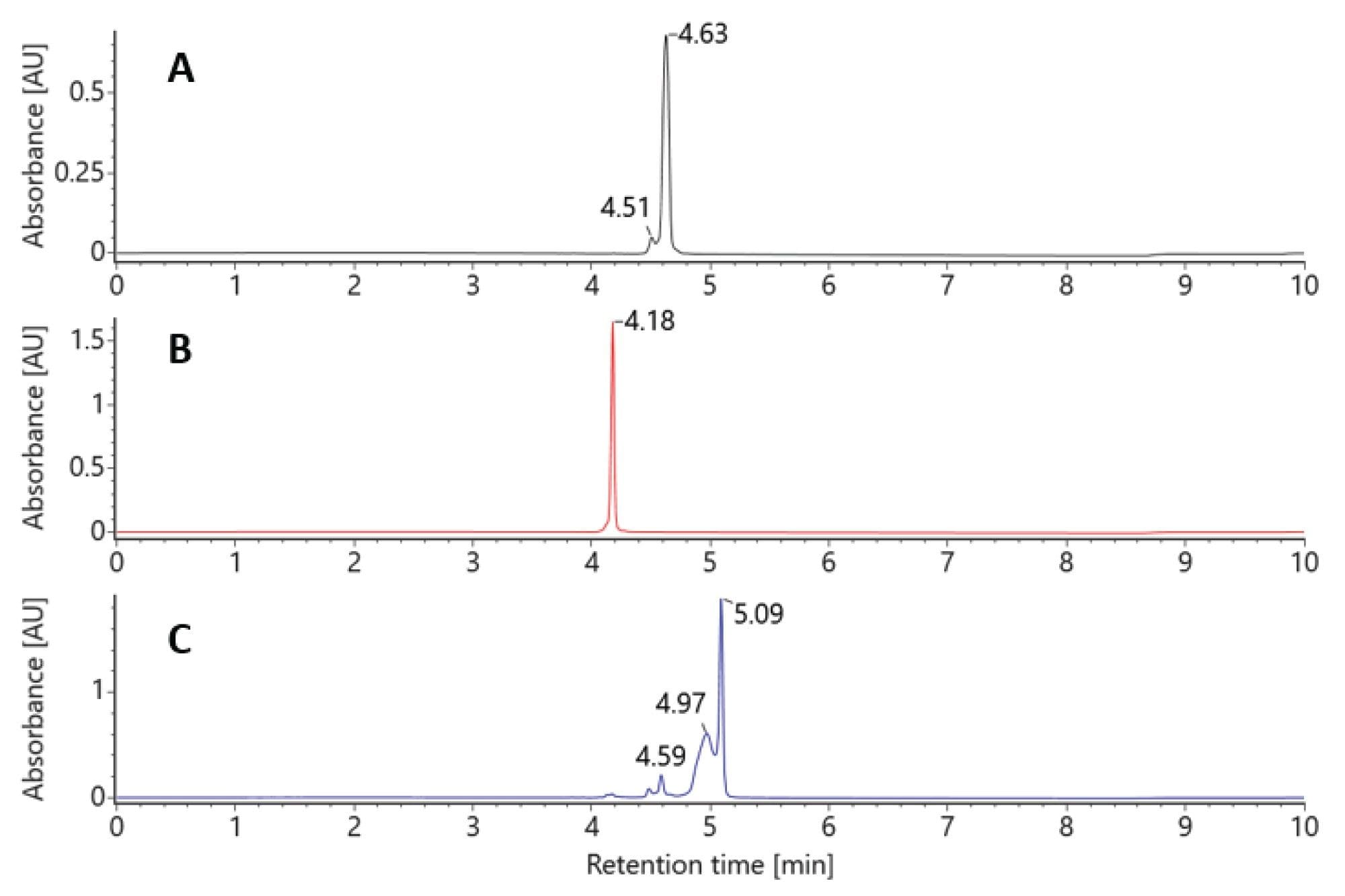
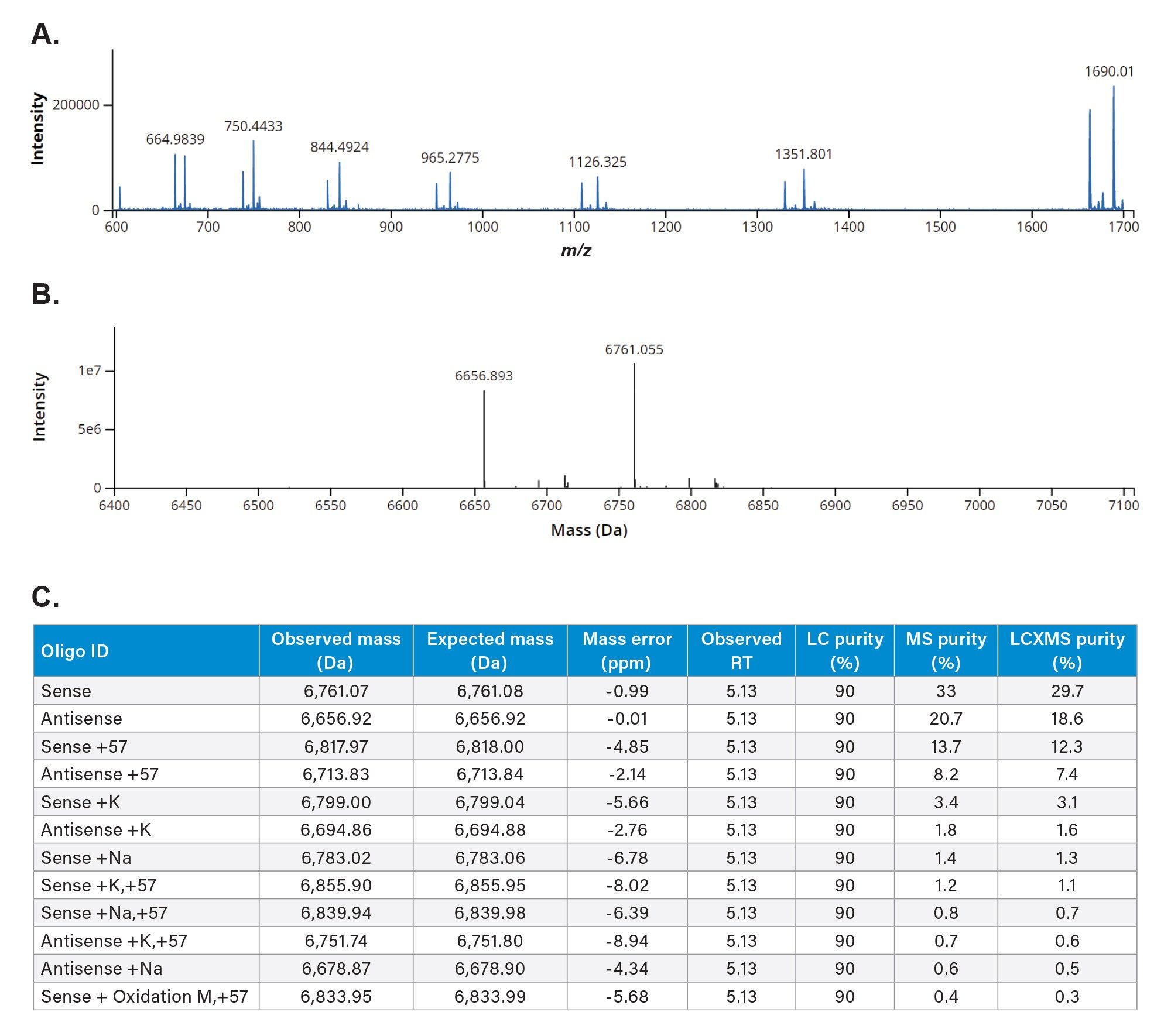
Figure 3A. Negative Ion ESI raw spectra the duplex siRNA dissociated during the electrospray process showing individual sense and antisense strand charge state envelopes eluting under the duplex peak at an LC retention time of 5.09 minutes.
B. waters_connect Intact Mass BayesSpray deconvoluted spectra of the duplex siRNA dissociated to sense and antisense strands during the electrospray process.
C. Results from waters_connect INTACT Mass App data processing of the dissociated duplex siRNA peak.
At an elevated column temperature of 60 °C the two pure standard oligos were once again chromatographically baseline separated. The duplex molecule was denatured on column, producing two chromatographically resolved single strands with retention times matching those of the individual standards (Figure 4).
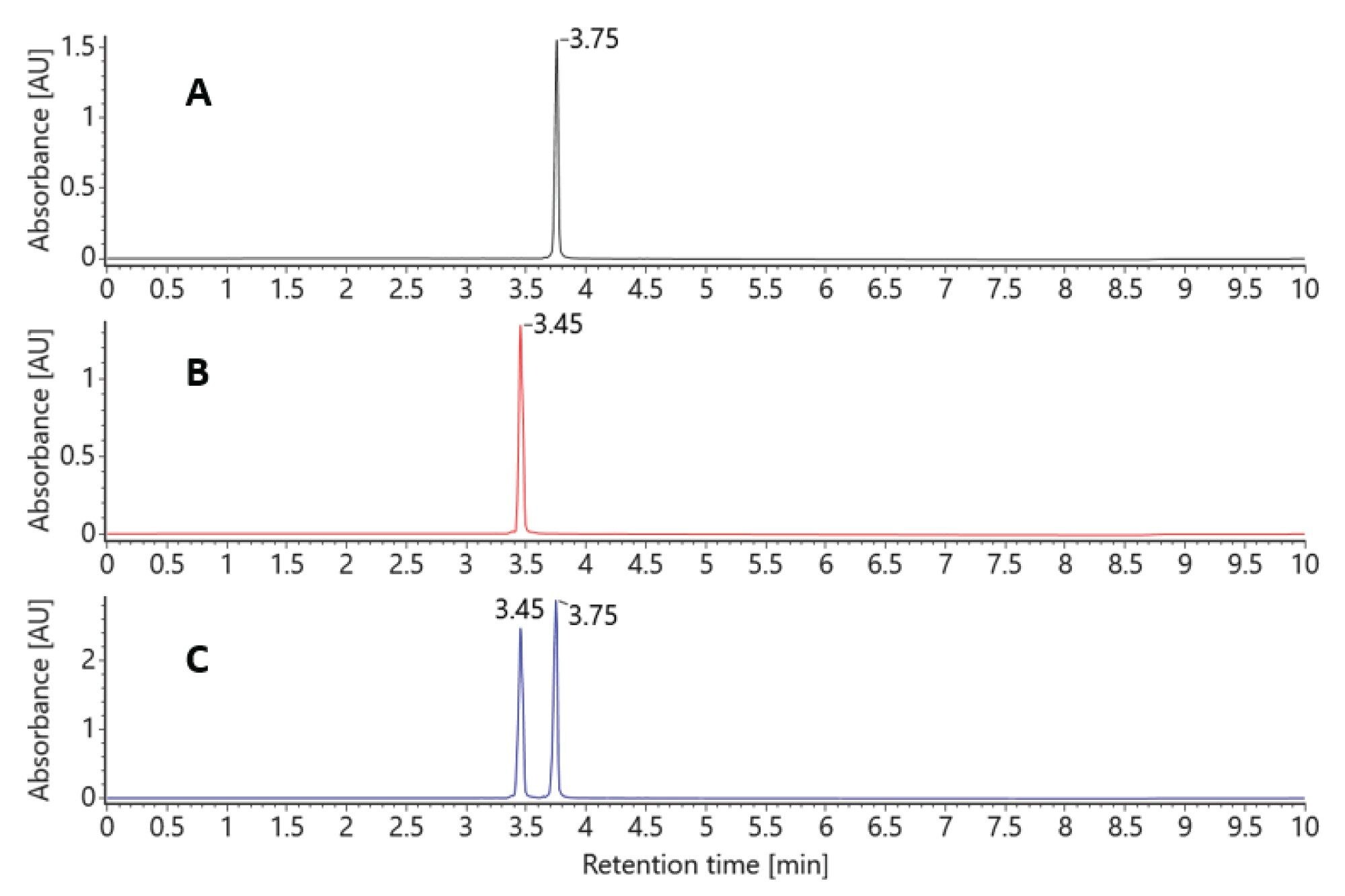
Both methods (denaturing and nondenaturing separations) with mass detection can be used to confirm the identity of the duplex siRNA by simply controlling the column temperatures and applying an automated data processing method.
The INTACT Mass processed data enabled rapid ID confirmation, but other informatics tools are required to support full sequence confirmation. The CONFIRM Sequence App can utilize the same BioAccord MSE full scan with fragmentation acquisition mode data to automatically confirm that the fragmentation data corresponds to the specified sequence.
Here, data from both siRNA single strands (nondenatured and co-eluting) were used to confirm the duplex sequence, achieving 90% sequence coverage of both strands using a voltage gradient from 100–120 V for the elevated energy fragmentation cycle. The unassigned region representing ~10% of the sequence coverage was further investigated, with chimeric spectral interferences from the fragments of the antisense strand identified as a reason for the missed assignments (Figure 5).
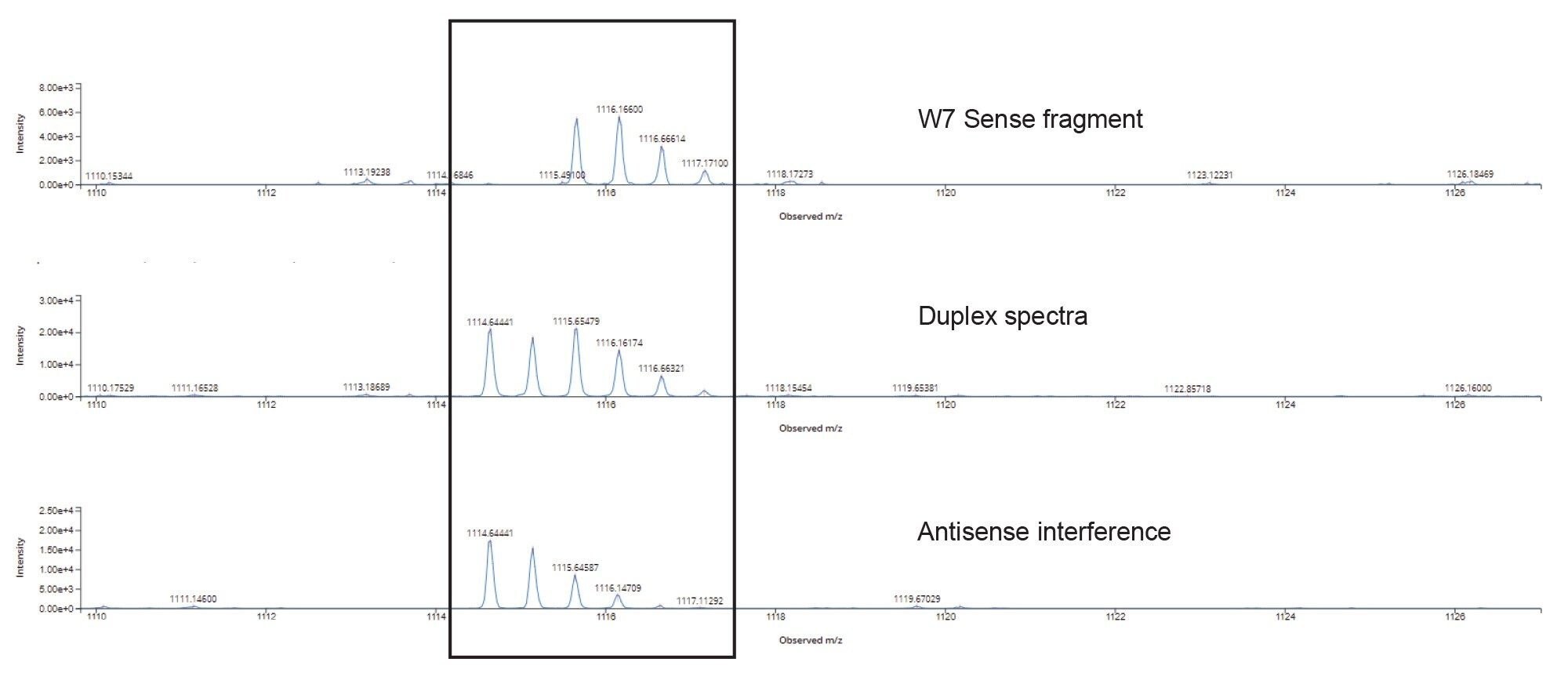
Overlapping isotopic patterns in the MSE fragmentation data from the 1114.7 m/z Antisense w7 fragment (Figure 5) complicate the automatic assignment of the w7 Sense fragment as seen in the chimeric duplex spectra from the combined fragmentation of both co-eluting stands.
The siRNA duplex single strands were also analyzed with a targeted MS/MS approach (individually selecting specific charges states from each strand for fragmentation) on a Vion IMS-QTof MS system, which provided 100% sequence coverage on both strands. This targeted MS/MS approach can result in cleaner fragmentation spectra for each single strand, removing many of the interferences, and thereby facilitating full sequence coverage when chromatographic separation of the duplex was not obtained with lower column temperature separations.
The investigation and experiments at 60 °C elevated column temperature resulted in denaturing chromatographic conditions, causing the duplex to chromatographically separate into its single strand components. As the single strands are well resolved on the column with excellent peak shape, their MSE elevated energy fragment patterns are obtained independent of one another, preventing the overlapping isotopic species observed when the duplex was maintained on the column.
With the interferences removed, the 60 °C column temperature denaturing method now allows for 100% sequence coverage to be assigned using the BioAccord System MSE data-independent approach (Figure 7). Thus, this combined denaturing/non-denaturing methodology enables the investigation of duplex purity, duplex identity, and single strand identity in a single acquisition. Sequence information can be obtained by tandem MS/MS on a QTof system but also on the BioAccord LC-MS System using a non-targeted data-independent approach.
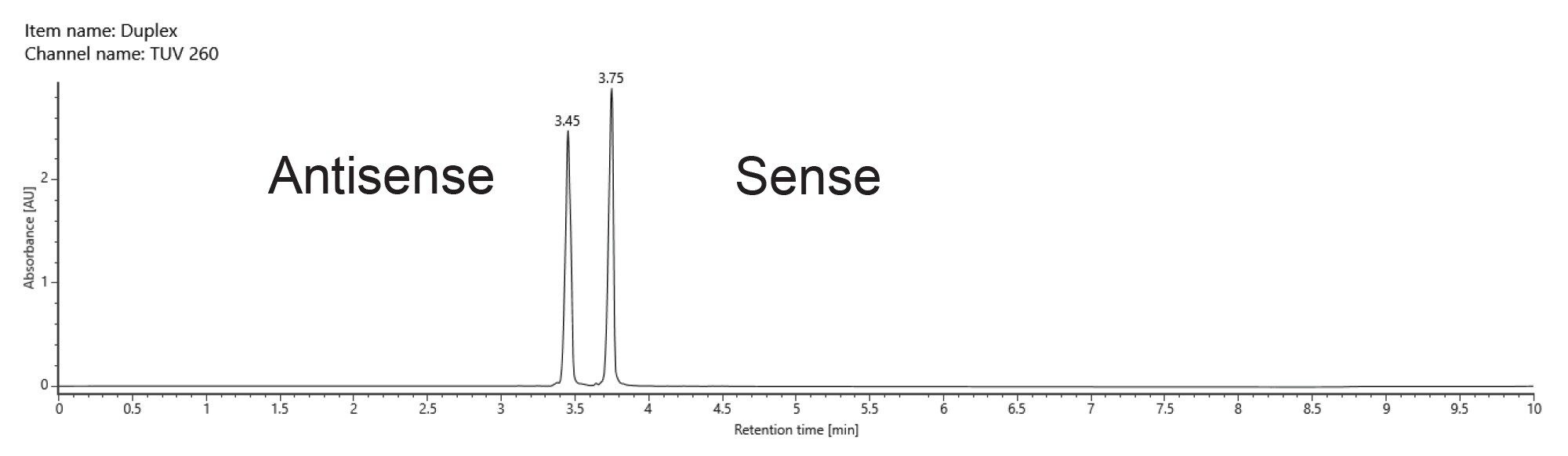
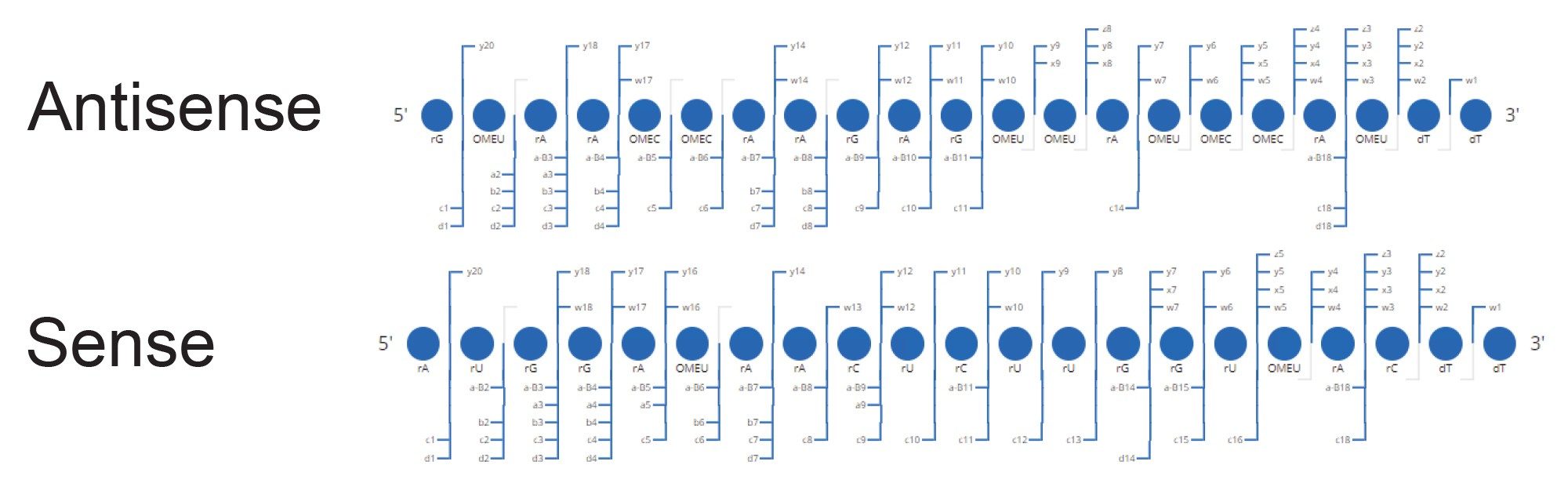
Additional experiments were conducted to ensure that elevated energy MSE spectra generated from the denatured duplex siRNA and pure single strand standards were comparable (Figure 8).
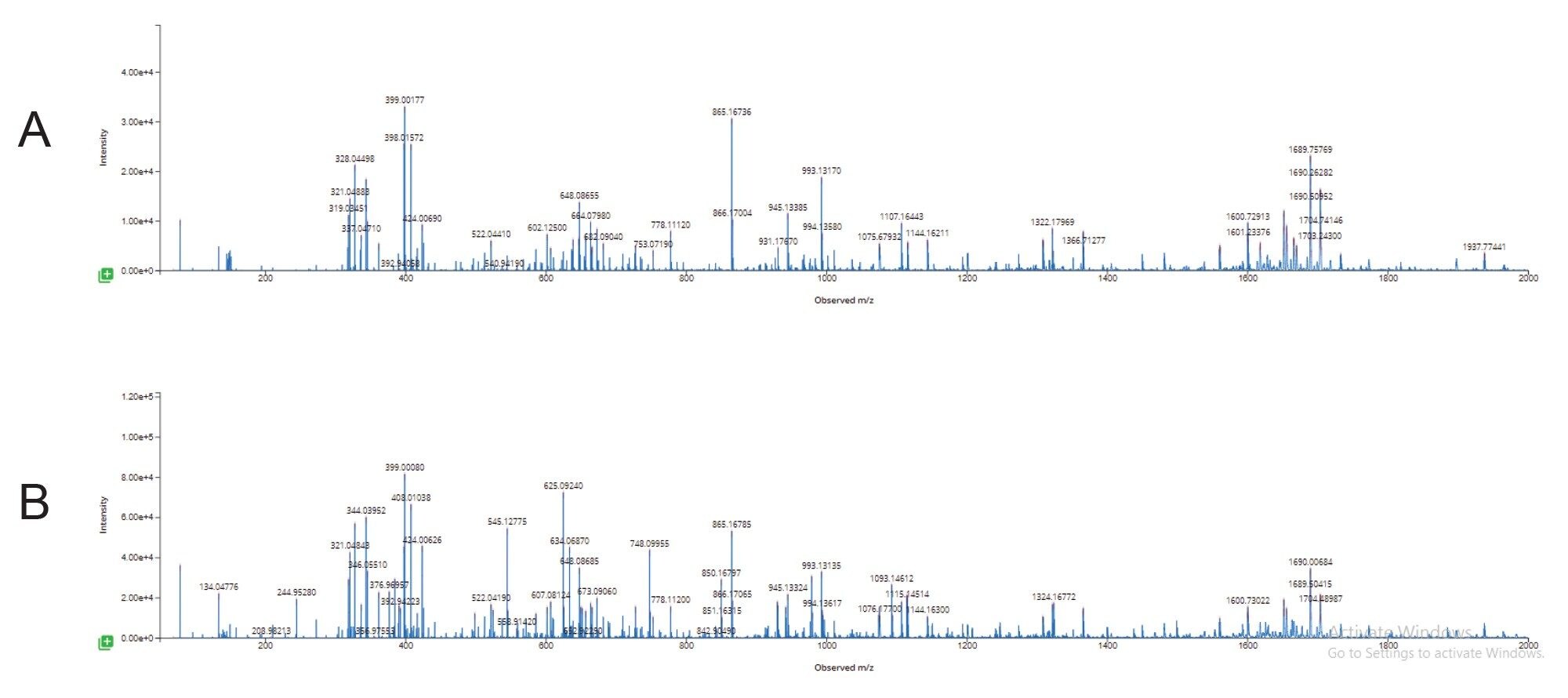
Conclusion
Denaturing (higher column temperature) and non-denaturing (lower column temperature) LC-MS methods have been developed that can assess the single strand purity, single strand identity, duplex purity and duplex identity of a duplex siRNA molecule using TOF or a QTof MS detection.
These methods made use of the waters_connect INTACT Mass and CONFIRM sequence Apps provide data analysis automation for intact mass and fragmentation based sequencing analysis.
Complete sequencing was obtained for both siRNA strands on the BioAccord LC-MS System using a data-independent MS fragmentation approach, that benefited from the chromatographic separation obtained in the denaturing LC separation.
Complete sequencing was obtained for both siRNA strands on the Vion IMS-QTof system by targeted MS/MS that was more tolerant of oligonucleotide strand co-elution in the non-denaturing LC separation.
The workflows explored in this application note show the complementary nature of the use of non-denaturing and denaturing duplex siRNA analysis, and the ability to make use of more complex QTof instrumentation in a development environment, and the more fit-for-purpose BioAccord LC-MS System for routine analysis in development or for validatable assays in regulated manufacturing and quality organizations.
References
- Al Shaer D, Al Musaimi O, Albericio F, de la Torre BG. 2021 FDA TIDES (Peptides and Oligonucleotides) Harvest. Pharmaceuticals (Basel). 2022;15(2):222. doi:10.3390/ph15020222
- Al Musaimi O, Al Shaer D, Albericio F, de la Torre BG. 2022 FDA TIDES (Peptides and Oligonucleotides) Harvest. Pharmaceuticals. 2023;16(3):336. doi:10.3390/ph16030336
- Al Shaer D, Al Musaimi O, Albericio F, de la Torre BG. 2023 FDA TIDES (Peptides and Oligonucleotides) Harvest. Pharmaceuticals (Basel). 2024;17(2):243. doi:10.3390/ph17020243
- Rossi JJ, Rossi DJ. siRNA Drugs: Here to Stay. Molecular Therapy. 2021;29(2):431–432. doi:10.1016/j.ymthe.2021.01.015
- Gilar M, Koshel BM, Birdsall RE. Ion-Pair Reversed-Phase and Hydrophilic Interaction Chromatography Methods for Analysis of Phosphorothioate Oligonucleotides. Journal of Chromatography A. 2023;1712:464475. doi:10.1016/j.chroma.2023.464475
- Cook K, Thayer J. Advantages of Ion-Exchange Chromatography for Oligonucleotide Analysis. Bioanalysis. 2011;3(10):1109–1120. doi:10.4155/bio.11.66
- Shimoyama A, Fujisaka A, Obika S. Evaluation of Size-Exclusion Chromatography for the Analysis of Phosphorothioate Oligonucleotides. Journal of Pharmaceutical and Biomedical Analysis. 2017;136:55–65. doi:10.1016/j.jpba.2016.12.036
- Donegan M, Nguyen JM, Gilar M. Effect of Ion-Pairing Reagent Hydrophobicity on Liquid Chromatography and Mass Spectrometry Analysis of Oligonucleotides. Journal of Chromatography A. 2022;1666:462860. doi:10.1016/j.chroma.2022.462860
- Abdullah AM, Sommers C, Hawes J, Rodriguez JD, Yang K. Tandem mass spectrometric sequence characterization of synthetic thymidine-rich oligonucleotides. Journal of Mass Spectrometry. 2022;57(4):e4819. doi:10.1002/jms.4819
- Catalin E. Doneanu, Ying Qing Yu, Joseph Fredette, William J. Warren, Weibin Chen. HILIC as an Alternative Separation Mode for Intact Mass Confirmation of Oligonucleotides on the BioAccord System. Waters application note. 720007395. 2021.
Acknowledgement
We would like to acknowledge the contributions of Rebecca Hirschberger and Michael Rühl from BioSpring.
720008654, December 2024