Improved Bioanalytical Extraction of Therapeutic Antisense Oligonucleotides, Including a Lipid Conjugated ASO From Liver and Brain Tissue Using the OligoWorks™ SPE Microplate Kit
Abstract
To develop and optimize the sample preparation and extraction of therapeutically-relevant oligonucleotides, including lipid conjugated antisense oligonucleotides (ASOs), from liver and brain tissue using a Proteinase K facilitated sample digestion and homogenization procedure, followed by weak anion exchange (WAX) mixed-mode SPE to achieve high recoveries of target oligonucleotides.
Benefits
- High oligonucleotide tissue recovery (≥80%) and repeatability (SDs ≤10%) using a Proteinase K facilitated tissue homogenization protocol followed by mixed-mode SPE in a 96-well microelution plate format
- Low-volume and highly concentrated MS-compatible eluate
- Direct LC-MS injection of SPE eluate without having to evaporate and reconstitute
- Short LC-MS gradient ensuring high throughput analysis
- Improved recovery of lipid conjugated ASO from brain and liver tissue using NP-40 alternative non-ionic surfactant and MS-optimized SPE wash steps
Introduction
The field of oligonucleotide therapy is rapidly evolving to battle genetic disorders, cancers, and viral infections. Such therapies offer tissue-specific alteration of gene expression through customizable drugs with a wide range of applications and tunability. Thus, there is a growing need for their accurate quantitative measurement from biomatrices in support of drug metabolism and pharmacokinetic (DMPK) studies to assess drug efficacy and safety. Such measurements are challenging due to the complexity of tissue biomatrices and drug modifications that are being incorporated to enhance stability and delivery.
In this study, we present an optimized, kit-based bioanalytical workflow tailored to improve the extraction and recovery of oligonucleotides from various tissue matrices. Leveraging the OligoWorks SPE Microplate Kit with RapiZyme™ Proteinase K digestion module, we achieved highly reproducible analyte recovery: ≥80% for two phosphorothioated ASOs (GEM 91 and GEM132) as well as a lipid conjugated ASO (Waters Lipid ASO LC-MS Standard). Additionally, we discovered for the lipid-conjugated ASO in particular, adding a small amount of the non-ionic surfactant, NP-40 alternative to the tissue homogenization step, led to enhanced extraction from liver and brain tissue.
Experimental
LC Conditions
UPLC: |
ACQUITY UPLC H-Class PLUS System with FTN Sample Manager |
MPA: |
1% HFIP (Hexafluoro-2-propanol) 0.1% DIPEA (N,N-Diisopropylethylamine) in 99% Water |
MPB: |
0.75% HFIP 0.0375% DIPEA. 65% Acetonitrile (ACN), 35% Water |
Column/sorbents: |
ACQUITY Premier Oligonucleotide C18 Column, 130 Å, 1.7 µm 2.1 x 50 mm |
Col temperature: |
55 °C |
Sample temperature: |
15 °C |
Inj. Vol: |
5–15 µL |
Purge solvent: |
90:10 H20:MeOH (Methanol) |
Wash solvent: |
25:25:25:25 Water:MeOH:ACN:IPA |
MS Conditions
MS: |
Xevo TQ-Absolute |
Capillary (kV): |
0.5 |
Desolvation temperature: |
600 °C |
Desolvation flow: |
1000 L/Hr |
Cone Gas flow: |
150 L/Hr |
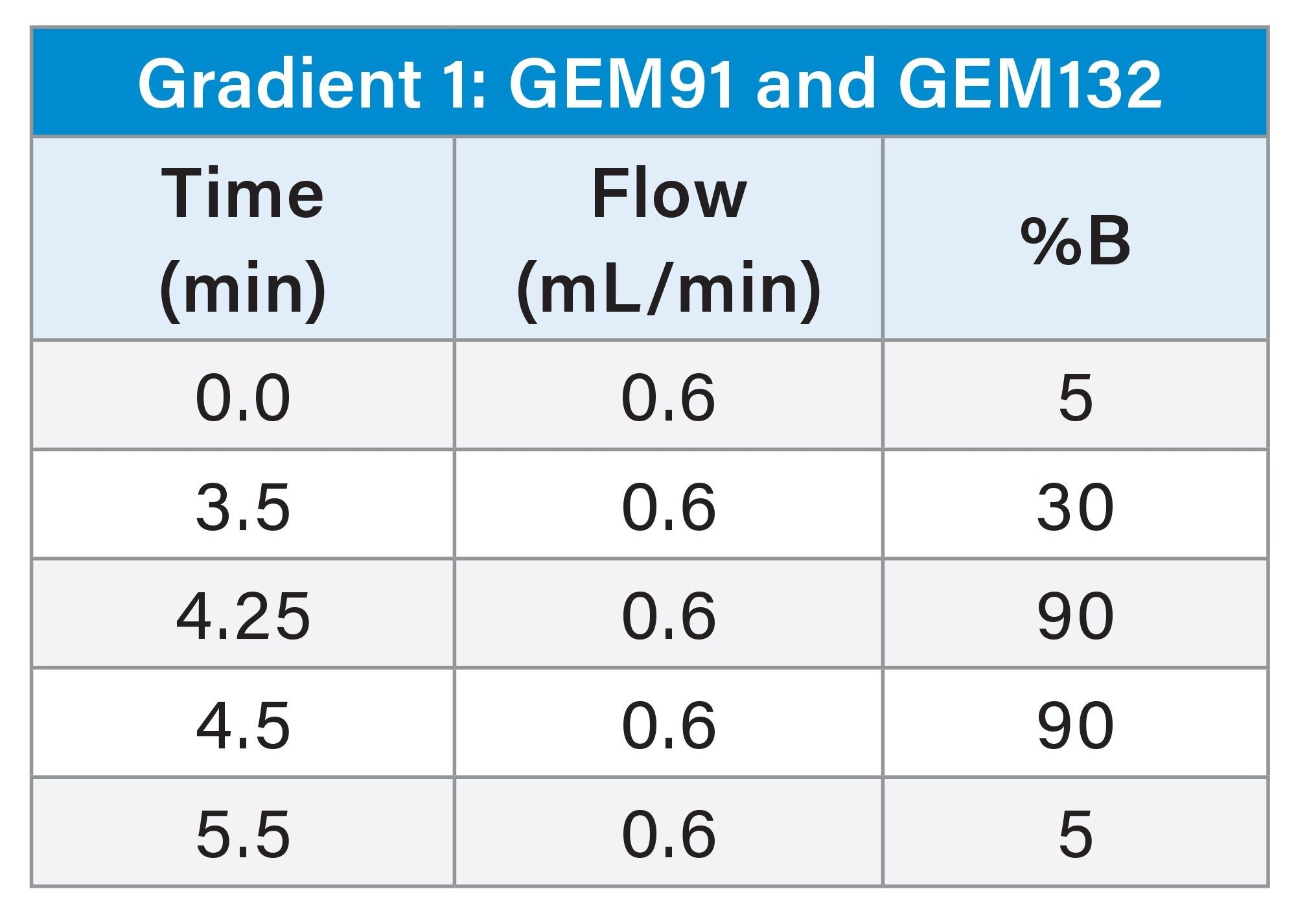
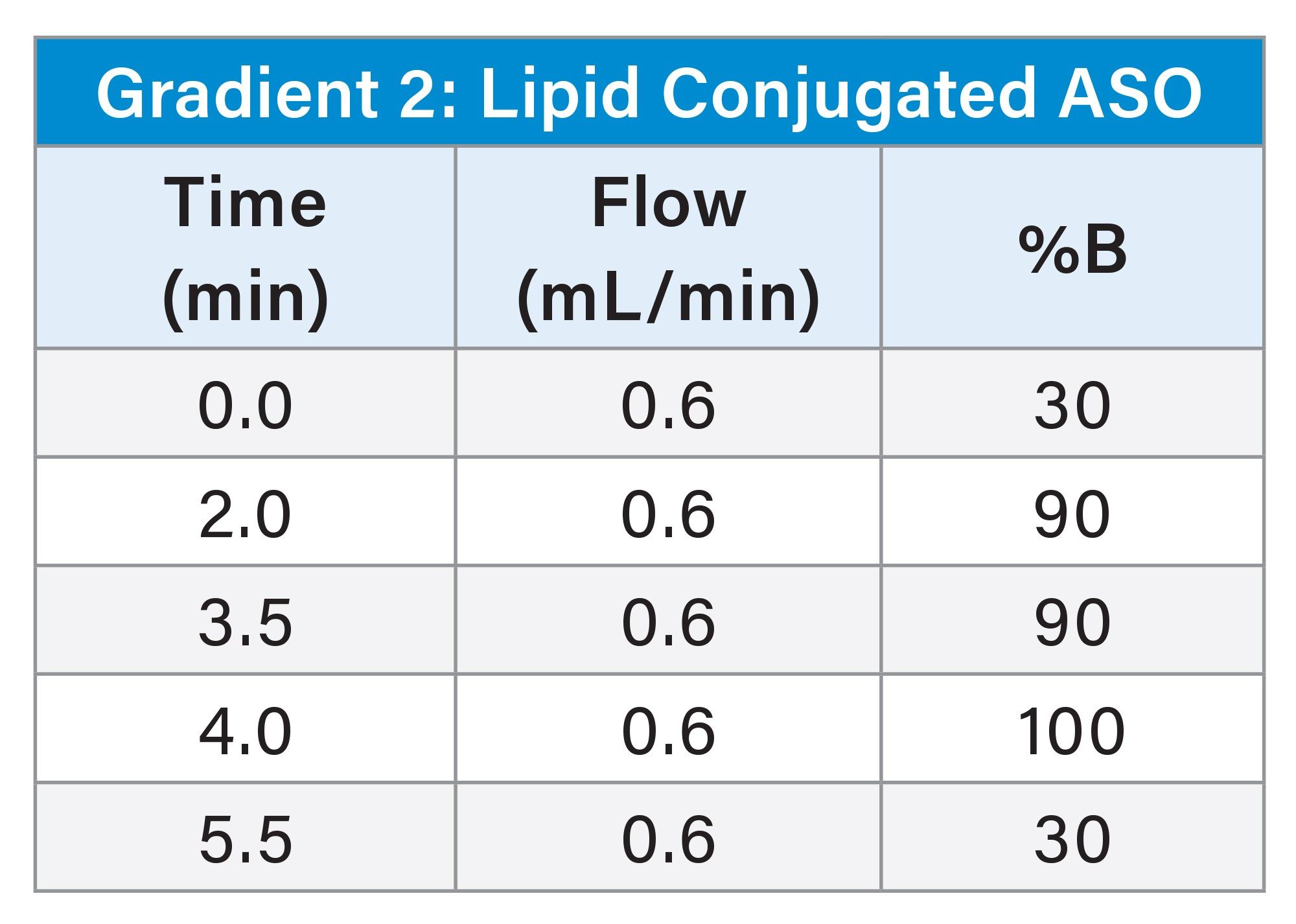
Sample Pretreatment and SPE Extraction
Chemicals, Reagents, Materials and Solvents
MS grade methanol, acetonitrile, and isopropanol, were purchased from Honeywell, Burdick Jackson. Hexafluoro-2-propanol (HFIP) was purchased from Oakwood Chemicals. N,N-Diisopropylethylamine (DIPEA) and ammonium acetate were purchased from Sigma Aldrich (St. Louis, MO, USA). In-house 18.2 Ω*cm purified water was used to prepare LC mobile phases. Triton™X-100 reduced nonionic surfactant ( p/n: 282103) and IGEPAL CA-630 non-denaturing detergent (p/n: 18896) were purchased from Sigma-Aldrich. NP-40 Alternative nonionic surfactant (p/n: 492016) was purchased from EMD Millipore. DNase/RNase-free distilled water and 1 M Ultrapure™ Tris-HCl solution (p/n: 15567–027) were purchased from Thermo Fisher Scientific. OligoWorks SPE Microplate Kits (p/n: 186010614) were procured from Waters Technologies Corporation. Porcine liver tissue was sourced from local market and mouse brain tissue was sourced from BIIOIVT Westbury, NY. Liver and brain tissue were stored 20 °C until sample processing and extraction. Tissues were homogenized using Precellys™ Lysing Kit, 2 mL tubes (P000918-LYSK0-A).
The oligonucleotides used in this assessment are described below.
- Gene expression modulator 91 (GEM 91), a 25-mer phosphorothioated antisense oligonucleotide (MW 7771), synthesized by Nitto Denko Avecia (Milford, MA)
- GEM 132, a 20-mer phosphorothioated antisense oligonucleotide with 2’ methoxy caps (MW 6600), synthesized by Nitto Denko Avecia (Milford, MA)
- The Waters Lipid Conjugated ASO LC-MS standard is a 16-mer antisense oligonucleotide with a 5’ palmitate modification, a phosphorothioated backbone and terminal methoxy ethyl modifications (MW 6050) (Waters p/n: 186010747)
Oligonucleotide Standard and Tissue Sample Preparation
The recovery of oligonucleotides from tissue was assessed according to the following procedure. GEM 91, GEM 132, and the lipid-conjugated ASO were prepared and extracted using the workflow and protocol shown in Figure 1. A 50 mg (±0.2 mg) quantity of tissue sample was weighed into a 2 mL lysis tube. A 20 µL aliquot oligonucleotide stock solution (consisting of 0.05 µg /µL concentrations of GEM 91, GEM 132, and the lipid-conjugated ASO prepared in 100 mM ammonium acetate pH 5.5 buffer) was added to the 50 mg of tissue.
To these oligonucleotide spiked tissue samples, 105 µL of a 1M Tris-HCl solution and 150 µL acetonitrile were added. The samples were then briefly vortexed, and the RapiZyme Digestion Module reagents (p/n: 186010601) were added. Specifically, 50 µL of a 6M GuHCl solution, 50 µL of a 0.5M TCEP HCl solution, and 125 µL of RapiZyme Proteinase K 20 mg/mL solution were added. The final tissue sample liquid volume with all reagents added was 500 µL, with a final (individual) oligonucleotide concentration of 0.002 µg /µL (2 µg/mL) solution.
This tissue mixture was then homogenized and digested using the details shown in Figure 1. Following sample homogenization/digestion, samples were clarified using cold centrifugation (9,400 RCF) for 30 minutes. This supernatant (100 µL) was subsequently purified using the OligoWorks SPE Microplate (p/n: 186010618) and OligoWorks SPE Eluent (p/n: 186010610). This SPE protocol is also listed in Figure 1. A QuanRecovery with MaxPeak High Performance Surfaces 700 µL 96-well Collection Plate was used to collect the SPE eluate fraction. This 50 µL eluate containing the extracted oligonucleotides was then diluted 1:1 with water, mixed, and subsequently analyzed by IP-RP LC-MS/MS using a 10 µL injection of the prepared and extracted sample.
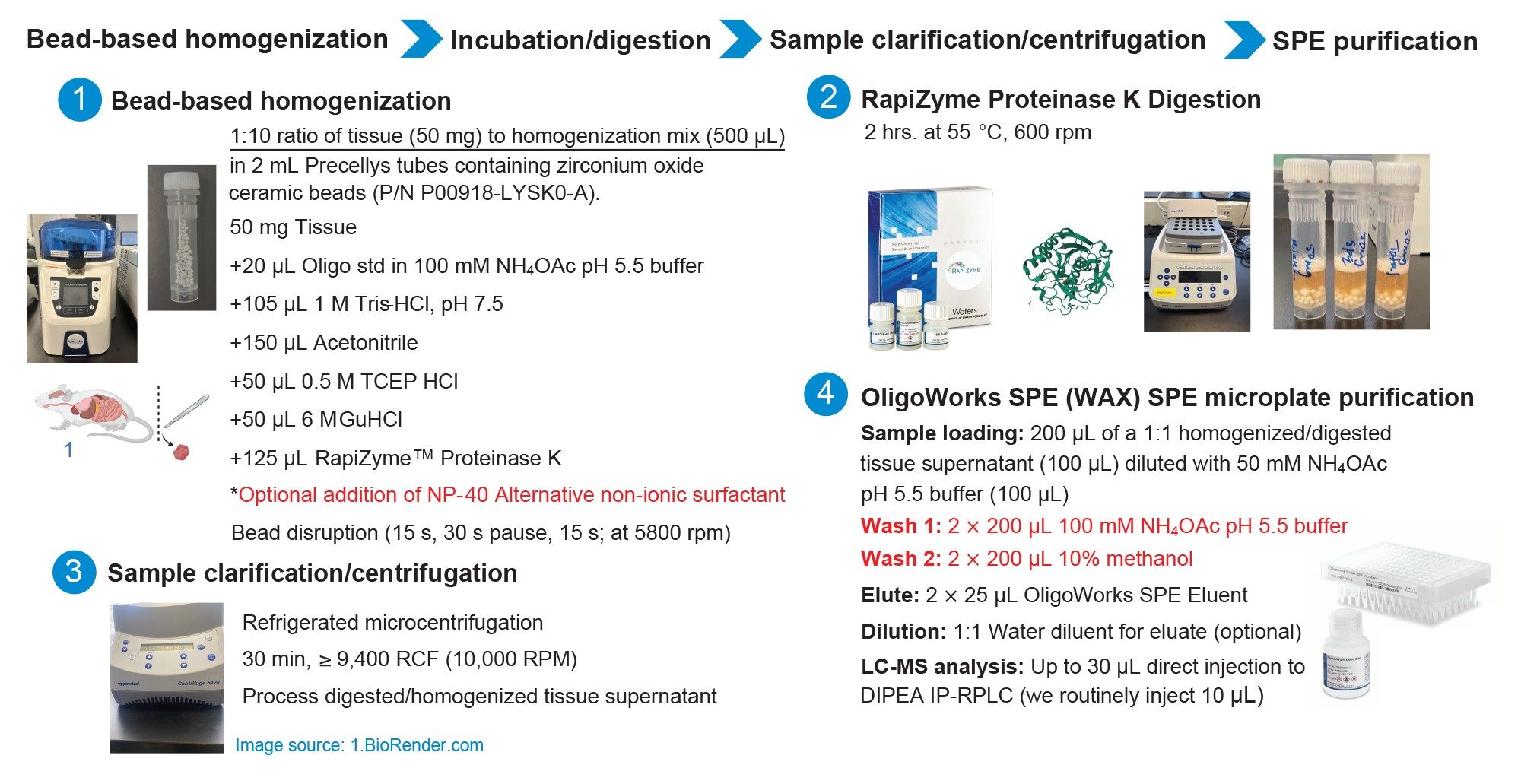
LC-MS Analysis
An ion-pairing, reversed-phase ultra-performance liquid chromatographic (UPLC) gradient separation was performed using an ACQUITY UPLC H-Class PLUS System with Quaternary Solvent Manager (QSM), configured with a Flow Through Needle (FTN) and an ACQUITY Premier Oligonucleotide BEH C18 130 Å, 1.7 µm Column (p/n: 186009484). The Xevo TQ Absolute Tandem/Triple Quadrupole Mass Spectrometer (MS) was used for oligonucleotide detection and quantitative analysis using multiple reaction monitoring (MRM). UPLC Gradient and MS conditions are listed in the experimental section. The MRM transitions used for detection and quantification for each oligonucleotide evaluated are shown in Table 1.
Note: an ACQUITY Premier LC System can be applied in this workflow to further improve precision and accuracy of the separation.
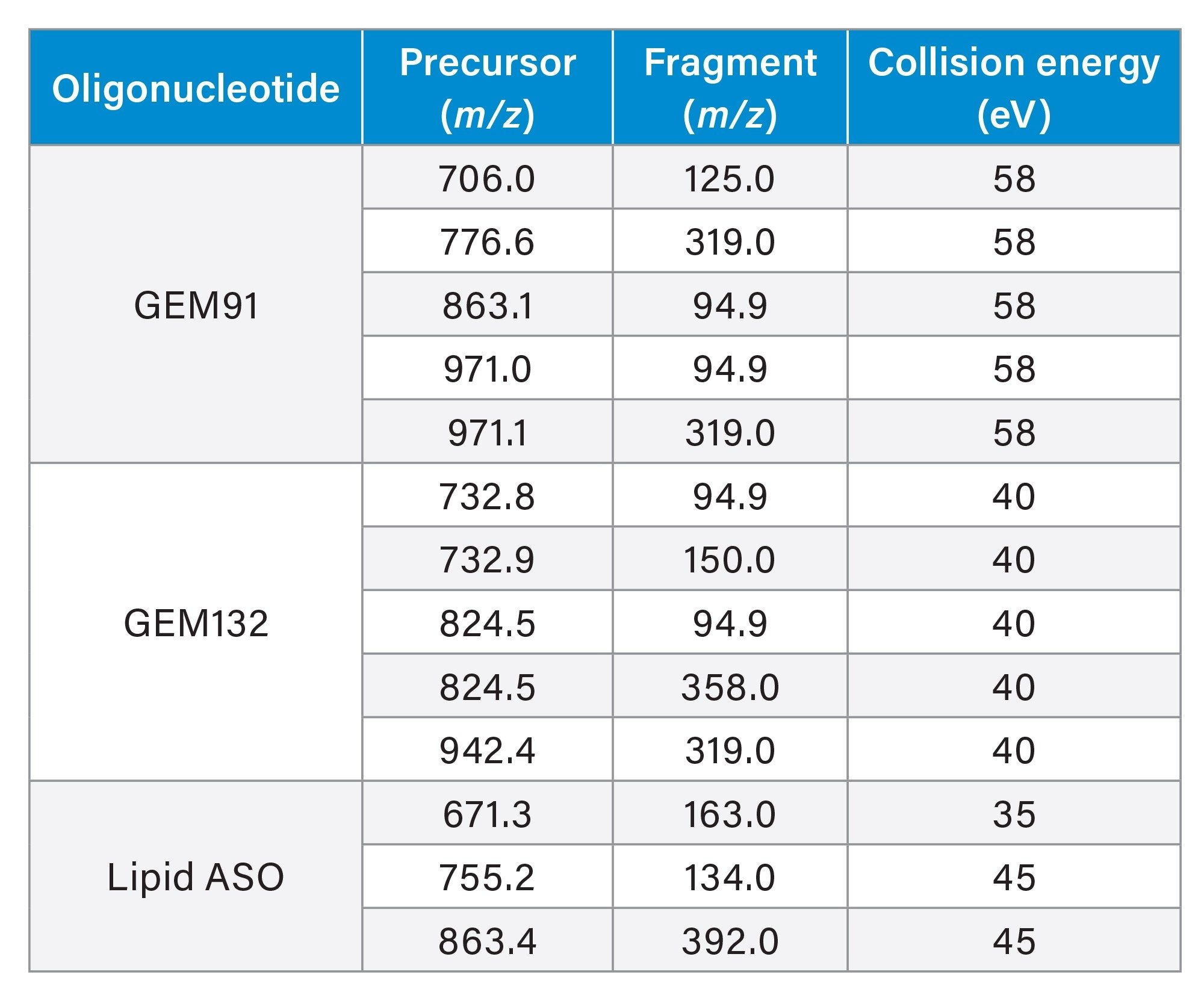
Results and Discussion
Developing bioanalytical LC-MS methods for oligonucleotide therapeutics presents significant challenges due to the complexity and variety of oligonucleotide structures, as well as their very low concentrations in biological samples. This often necessitates extensive sample preparation investigations to establish homogenization, extraction, and purification protocols for extraction of the oligonucleotides from biological matrices with high specificity and efficiency. These analytical methods must be sensitive, selective, and repeatable. The intricate nature of biological matrices, especially organ tissues rich in proteins and lipids, can significantly impede the accurate extraction and recovery of oligonucleotides due to strong protein binding. This challenge is further exacerbated when oligonucleotides are modified with residues and conjugate moieties that are designed to enhance their delivery and stability. Overcoming these obstacles is crucial for developing a robust and sensitive bioanalytical method for oligonucleotides.
Standard sample preparation techniques for processing tissues often utilize lysis buffers that contain non-ionic surfactants, as these surfactants effectively solubilize cell membranes without denaturing proteins, making them well-suited for nucleic acid extraction.1–4 However use of these buffers is not always directly compatible with downstream LC-MS analyses, and extensive sample clean-up can be required to remove buffer salts, detergents and surfactants which impede chromatographic separations and suppress MS signal limiting the analytical performance of the resulting assay.5–7 Additionally, one particular surfactant, Triton X-100 reduced surfactant has generated much attention due to its ability to form highly toxic degradation products raising environmental and health concerns.8–9
Earlier proof-of-concept studies showed that a detergent-free sample pretreatment method, coupled with solid-phase extraction (SPE), effectively recovered oligonucleotides (https://www.waters.com/content/dam/waters/en/app-notes/2018/720008270/720008270-en.pdf).10 This process involved solvent-assisted tissue homogenization and digestion of liver tissue using the RapiZyme Digestion Module reagents included in every OligoWorks SPE kit, followed by SPE of the resulting supernatant. Recoveries of ≥59% were reported for GEM91, GEM132, and a lipid-conjugated ASO. This method not only provided adequate oligonucleotide recovery but also eliminated the need for lysis buffers or surfactants, making it suitable for LC-MS applications.
In this study, we pursued additional investigations into the analytical performance of the solvent-assisted proteinase K tissue homogenization, digestion, and SPE extraction protocol, utilizing the OligoWorks SPE Microplate Kit and reagents to extract oligonucleotides from bovine liver as well as porcine and mouse brain tissues. Figure 2 illustrates oligonucleotide extraction performance from bovine liver tissue, wherein ≥80% recovery was achieved with excellent repeatability, as indicated by standard deviations (SDs) of ≤10% (panel A) and extraction linearities of ≥0.98 for GEM91, GEM 132, and the lipid-conjugated ASO from bovine liver (panel B). Figure 3 illustrates this equivalent performance for extractions from porcine brain tissue. Importantly, it should be noted that these results were achieved without the use of an internal standard.
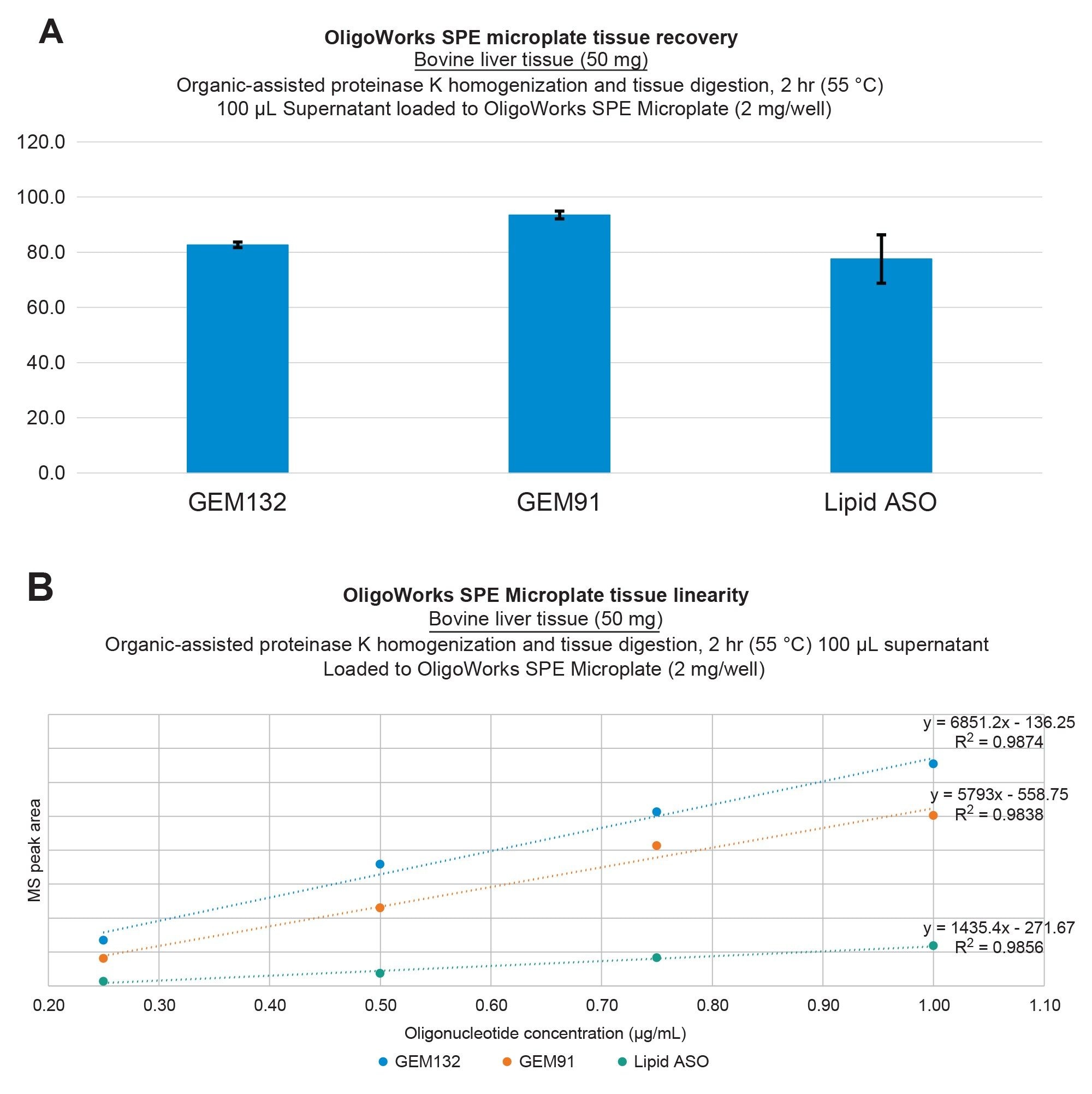
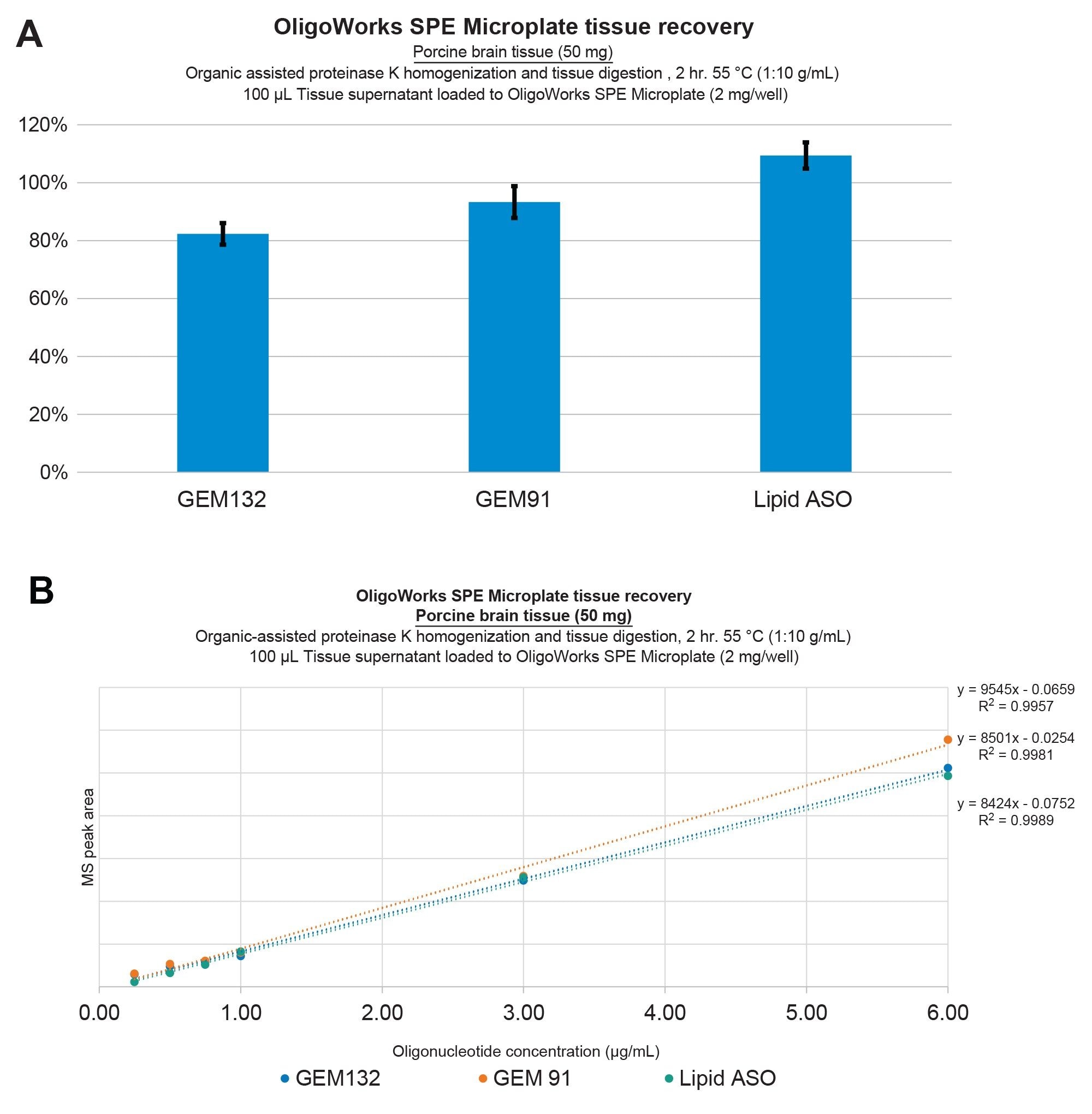
To enhance the recovery of the hydrophobic lipid-conjugated ASO, we focused on optimizing the digestion and the homogenization pretreatment step by incorporating non-ionic surfactants. Specifically, we studied the effects of NP-40 Alternative and IGEPAL CA-630 non-denaturing detergent, as well as the Triton X-100 reduced nonionic surfactant. Figure 4 provides the lipid conjugated ASO extraction performance from bovine liver tissue, demonstrating full recovery using the addition of 1% NP-40 Alternative vs 80% with no surfactant (panel A), while panel B demonstrates that low matrix effects were achieved. Incorporating NP-40 Alternative in the extraction of the lipid conjugated ASO from mouse brain tissue also improved its recovery from 20% (no surfactant) to 100% with the addition of a 2.5% concentration. This performance is shown in Figure 5. Upon using this surfactant, it is important to note that we also applied additional SPE wash steps, increasing them from a single 200 µL wash to two 200 µL washes of each wash solution. This adjustment was made to ensure thorough removal of these surfactants from the SPE sorbent bed before oligonucleotide elution was performed.
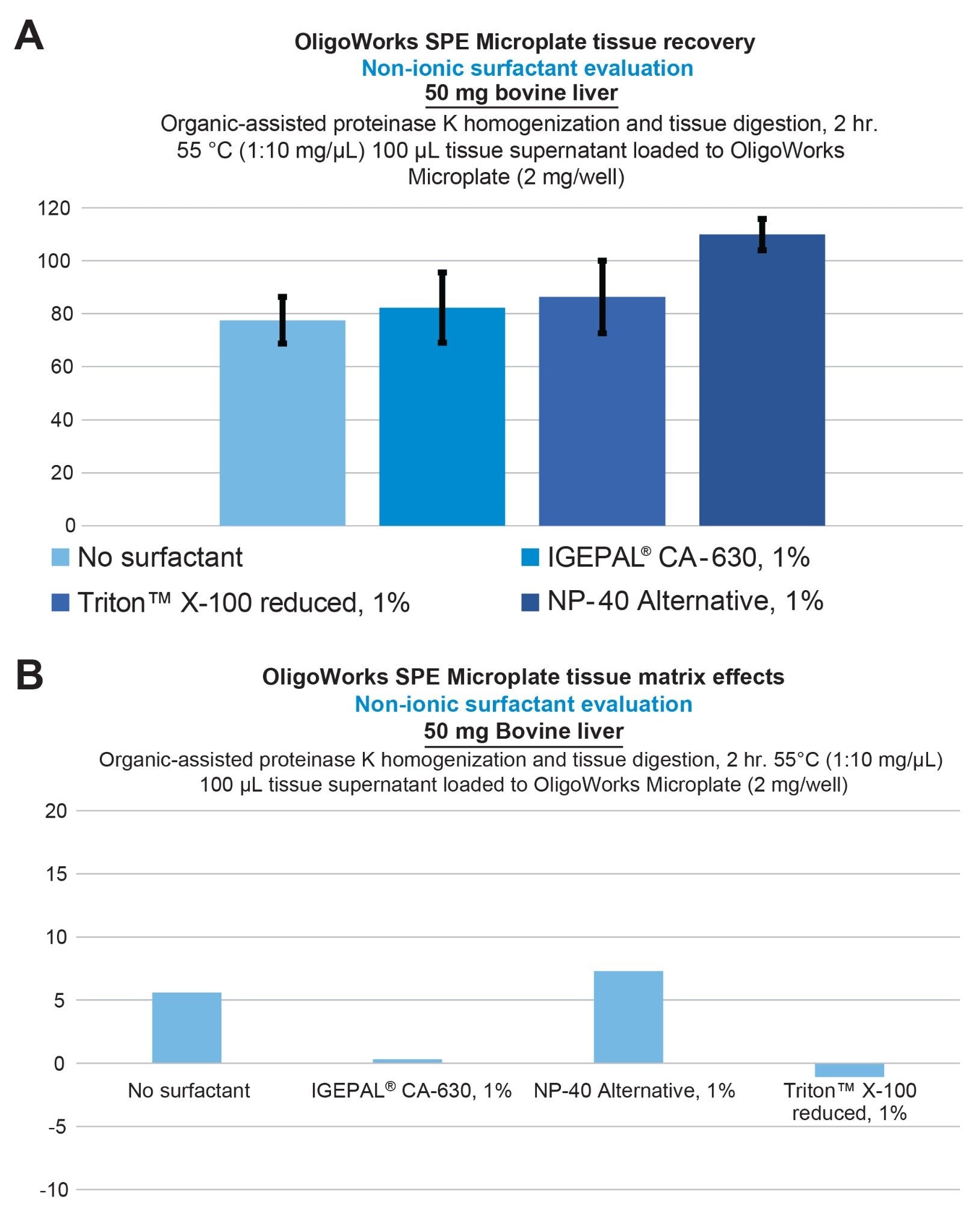
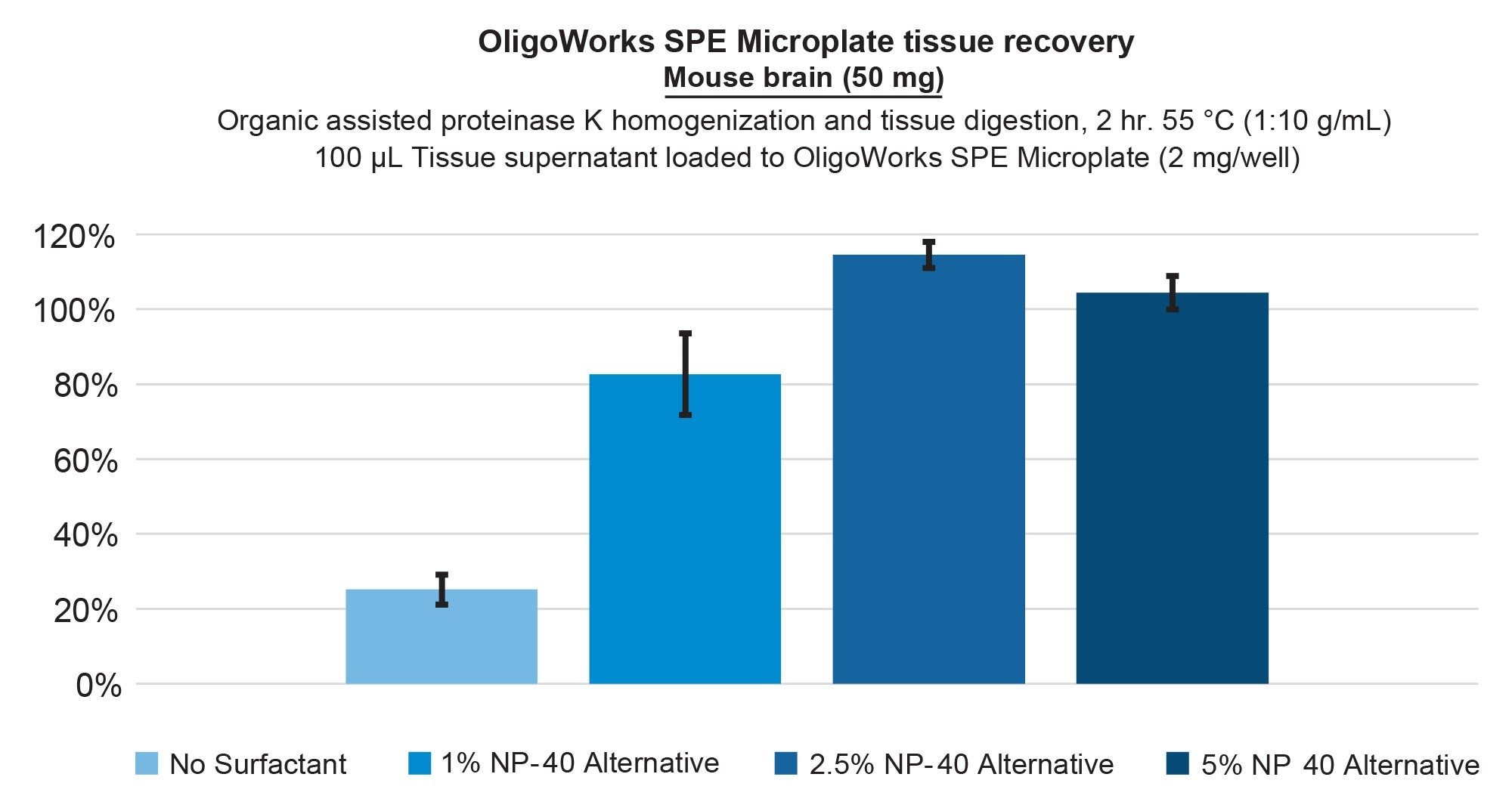
These findings demonstrate the applicability of the developed protocol for bioanalytical laboratories aiming to meet the growing demands of oligonucleotide therapeutic development. This optimized tissue method offers a practical, scalable solution for overcoming matrix-specific oligonucleotide recovery challenges, advancing the quantitative measurement of oligonucleotide therapeutics in drug development and clinical research.
Conclusion
This application effectively showcases an optimized, kit-based bioanalytical sample preparation workflow and its effectiveness at improving sensitivity and specificity in tissue analysis. By employing the OligoWorks SPE Microplate Kit containing the RapiZyme Proteinase K digestion module, we achieved highly reproducible analyte recoveries of ≥80% for GEM91 and GEM132 oligonucleotides and the Waters Lipid Conjugated ASO LC-MS Standard, which could be further enhanced through the optional use of NP-40 alternative non-ionic surfactant. Once optimized, this protocol was shown to yield complete recovery of the oligonucleotide analytes, whether extracted from liver or brain tissue. This optimized method offers a practical and scalable approach to address matrix-specific challenges. It is hoped that this work will be of assistance to analysts advancing the quantitative measurement of oligonucleotide therapeutics in drug development and clinical research.
OligoWorks, RapiZyme, QuanRecovery, MaxPeak, BEH, ACQUITY, UPLC, and Xevo are trademarks of Waters Technologies Corporation. Triton is a trademark of Union Carbide Corpration. IGEPAL is a trademark of Rhodia Operations. Precellys is a trademark of Bertin Technologies. All other trademarks are the property of their respective owners.
References
- Sambrook, J., & Russell, D. W. (2001). Molecular Cloning: A Laboratory Manual (3rd ed.). Cold Spring Harbor Laboratory Press.
- Boehm, M., & Guldbrandtsen, B. (1994). "Non-ionic surfactants for solubilization and stabilization of proteins." Methods in Enzymology, 227, 284–300.
- Kleiner, D. E., & Lentz, T. (2000). "Optimizing the extraction of RNA from tissues." BioTechniques, 29(1), 186–189. This article details methods for RNA extraction that include non-ionic surfactants.
- Gibson, T. J. (1995). "A Survey of Non-Ionic Surfactants." Trends in Biochemical Sciences, 20(8), 338–340.
- Roussel, C. et al. (2013). "Influence of lysis buffer composition on mass spectrometry-based proteomic analyses." Journal of Proteomics, 80, 78–90.
- Käll, L. et al. (2007). "Non-linear peptide ionization in nanoelectrospray ionization mass spectrometry." Molecular & Cellular Proteomics, 6(4), 555–565.
- McLafferty, F. W. et al. (2001). "Matrix effects in mass spectrometry: A review." Journal of Mass Spectrometry, 36(10), 1013–1020.
- Fent, K. et al. (2006). "Ecotoxicology of surfactants." Environmental Science & Technology, 40(7), 2243–2250.
- Baker, C. et al. (2017). "Surfactants and their effects on human health and the environment." Environmental Health Perspectives, 125(6), 064001.
- Van Tran, K., Trudeau M, and Lauber, M. High Oligonucleotide Recovery From Liver Tissue. Waters Application Note. 720008270. April, 2024.
720008656, January 2025